MINI-REVIEW
APOL1 Mediated Kidney Disease: A Review And Look Towards The Future
Authors
Vinay Srinivasan1 MD, Paolo Nikolai So2 MD, Edward P.K. Kwakyi3 MB ChB, Edgar V. Lerma4 MD, Nasim Wiegley5 MD
Affiliations
- Division of Nephrology, Cooper University Hospital and Cooper Medical School of Rowan University, Camden, New Jersey, USA
- Internal Medicine, Piedmont Athens Regional, Athens, Georgia, USA
- Department of Medicine and Therapeutics, University of Ghana Medical School, Accra, Ghana
- Section of Nephrology, University of illinois at Chicago, Chicago, Illinois, USA; Section of Nephrology, Advocate Christ Medical Center, Oak lawn, Illinois, USA
- Division of Nephrology, University of California Davis School of Medicine, Sacramento, California, USA
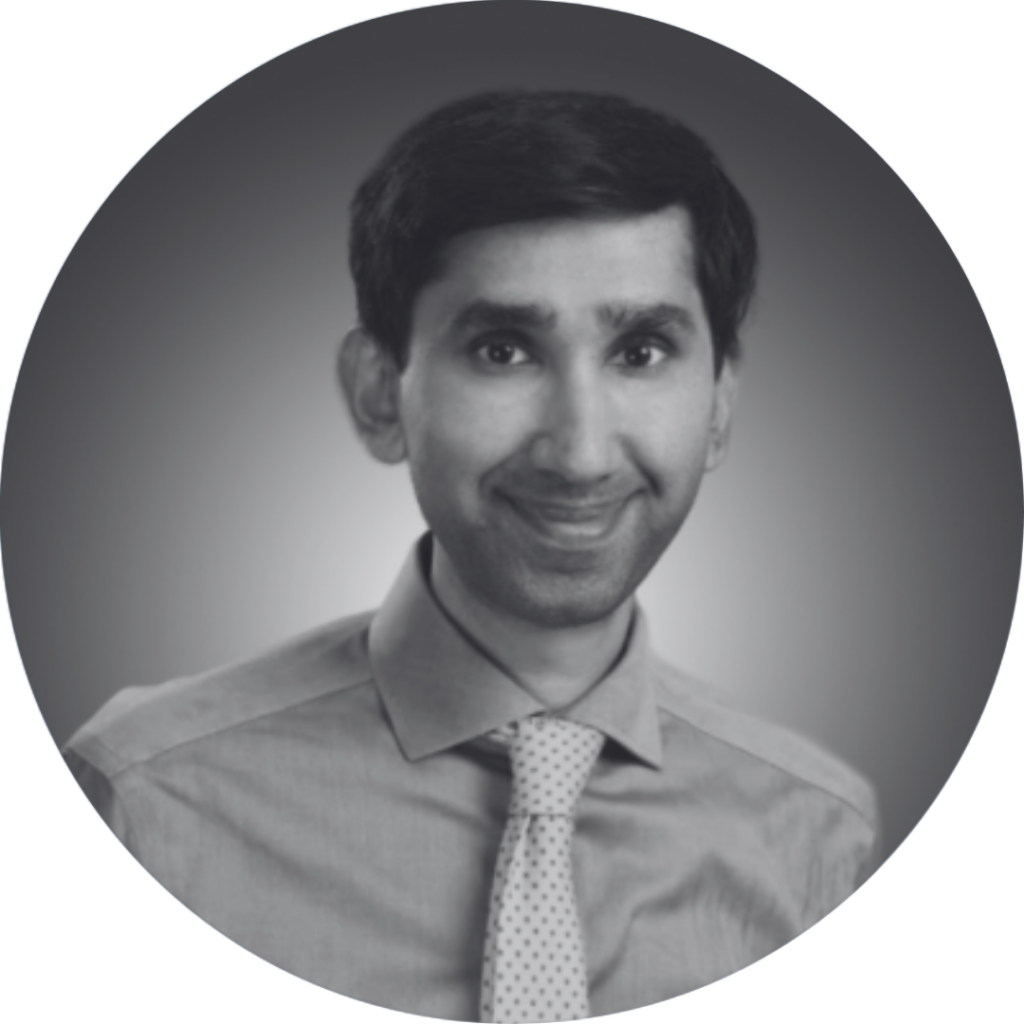
By Dr. Vinay Srinivasan
Assistant Professor of Medicine
Director of Onconephrology
Cooper University Hospital and
Cooper Medical School of Rowan University
New Jersey, USA
Abstract
Individuals of recent African ancestry are disproportionately affected by kidney disease. The discovery of the APOL1 gene and, subsequently, the G1 and G2 risk alleles has helped to understand some of these disparities. APOL1 does not appear to be necessary for normal kidney function, and the high-risk alleles appear to be gain of function mutations offering protection against Trypanosoma species. In the United States, approximately 6 million African Americans have a high-risk genotype. However, not all patients with high-risk genotypes will develop kidney disease, leading to the idea of a second hit hypothesis by certain genetic, environmental, and inflammatory triggers. Recent clinical trials have focused on the different postulated mechanisms of cellular toxicity, and one promising candidate, Inaxaplin, has advanced to a Phase 3 study. This review discusses the development of APOL1 risk alleles, postulated mechanisms of toxicity, and current targeted therapeutics given the worldwide burden of APOL1 mediated kidney disease.
Background
African Americans are disproportionately diagnosed with chronic kidney disease (CKD) and end-stage kidney disease (ESKD); in 2021, the prevalence of ESKD was over four times greater in black individuals compared to white individuals.1 Numerous factors, including genetic variants, account for this sharp discrepancy. In 2008, two individual groups reported genetic variants on human chromosome 22 that were linked to the greater incidence of non-diabetic kidney disease among African Americans, with the source eventually localized to the Apolipoprotein L1 (APOL1) gene that encodes apolipoprotein L1.2
APOL1 Genetics
APOL1 is absent among non-primates and has only been identified in a few higher order primates. The non-risk allele is identified as G0, and the two risk variants identified in 2010 are “G1” and “G2”. The G1 risk allele contains two amino acid substitutions, S342G and I384M, while the G2 risk allele has a two amino acid deletion (del388N389Y).2 Inheritance of these risk alleles follows a recessive pattern; the presence of one risk allele is associated with a small increase in the risk of kidney disease, while two risk alleles (G1/G1, G1/G2, or G2/G2) yield a high-risk genotype and, therefore a greater risk of kidney disease (Figure 1).3
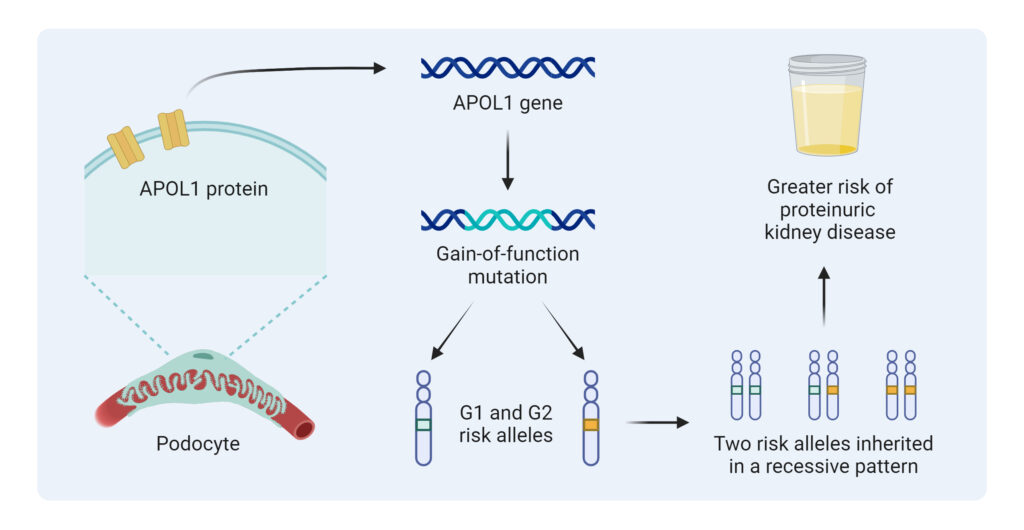
Figure 1: APOL1 High-Risk Variants Inheritance
Apolipoprotein L1 functions as a trypanolytic protein that lyses the species in animals; however, two infective subspecies, Trypanosoma brucei rhodesiense and Trypanosoma brucei gambiense, developed resistance mechanisms. Trypanosoma brucei rhodesiense generally causes acute sleeping sickness in East Africa, while Trypanosoma brucei gambiense causes chronic sleeping sickness in West Africa. The risk alleles, however, have been noted to have protective effects against these subspecies. The G1 risk allele appears to promote an asymptomatic carrier state to Trypanosoma brucei gambiense infection, while the G2 risk allele confers significant protection against Trypanosoma brucei rhodesiense infection.4
APOL1 circulates in the blood at high levels as part of a high-density lipoprotein (HDL) complex but is also found in peripheral tissue such as the kidney and lungs.5 However, circulating APOL1 levels have failed to correlate with kidney phenotypes in most studies, and kidney-associated APOL1 risk variants correlate with the development of kidney disease. This is supported by the fact that the risk of allograft loss after deceased donor kidney transplantation appears to increase for those who receive kidneys from high-risk APOL1 genotype donors.
Interestingly, APOL1 does not appear to be necessary for normal kidney function, as illustrated by the fact that an individual null for APOL1 in rural India did not have evidence of glomerulosclerosis. This individual came to attention after developing a Trypanosoma Evansi infection, a trypanosome endemic to water buffalos but against which humans have innate immunity due to APOL1 activity.6 While most recessive mutations are loss of function, APOL1 risk variants unusually appear to be gain of function mutations. They are not essential for normal kidney function but can increase the risk of developing kidney disease.2
Worldwide Burden of APOL1 Mediated Kidney Disease
The G1 and G2 risk alleles are most prevalent in West Africa, including the countries of Nigeria and Ghana, where the Yoruba and Igbo people of southwestern Nigeria have an APOL1 high-risk variant prevalence of approximately 40%. East African countries, however, appear to have the lowest prevalence of high-risk variants among African populations.7
Although the risk variants are found exclusively on chromosomes of those with recent African ancestry, they have been noted in other populations worldwide due to migratory patterns and the global African diaspora. In the United States, it is estimated that approximately 13% of African Americans, 6 million people, carry two high-risk alleles.7 Other populations, including those who identify as Hispanic, especially from Caribbean regions, have been noted to have an increased frequency of APOL1 risk alleles as well (Figure 2).8
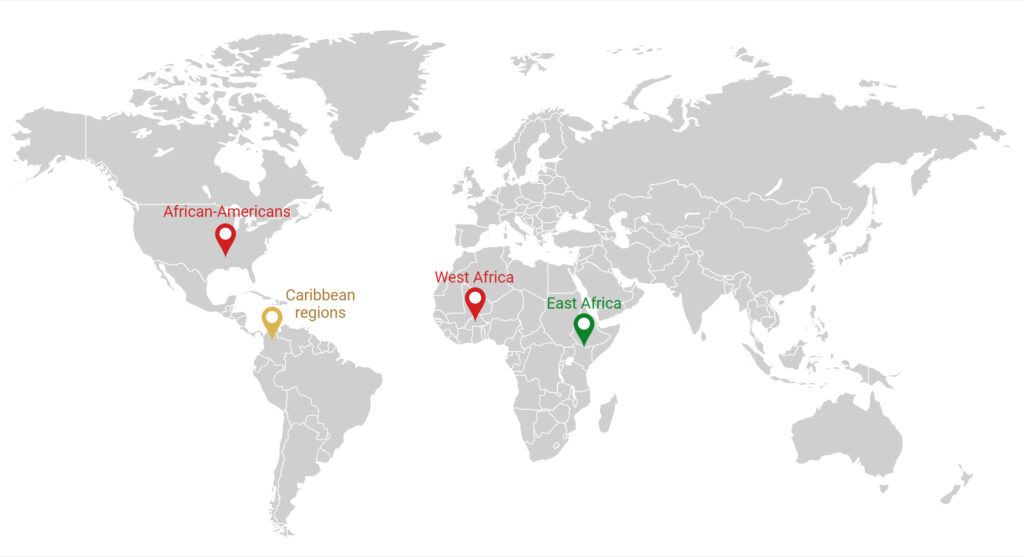
Figure 2: Global Distribution of APOL1 High-Risk Variants
Mechanism of Action
Numerous theories have been postulated regarding the way APOL1 risk variants cause nephrotoxicity. APOL1 has been shown to act as an ion channel in the lysosomal membranes of parasites and human cells although studies have shown conflicting results, whether this is due to a cation or anion channel depending on the pH and lipid environment. Other studies have also shown that APOL1 causes mitochondrial toxicity and increased stress to the endoplasmic reticulum.9
Podocytes that express APOL1 G1 and G2 risk alleles have been shown to increase the transcription of proteins, resulting in the formation of the inflammasome complex, ultimately resulting in increased Interleukin (IL)-1 and IL-18 transcription.10 Another study, however, proposed that podocytes derived from individuals with high-risk APOL1 variants have a significant reduction in Golgi phosphatidylinositol-4-phosphate [PI(4)P] levels, resulting in cellular reorganization.11 Ongoing research will assist with delineating these possible mechanisms of toxicity.
“Second Hit” Hypothesis
Although a significant minority of many populations have a high-risk APOL1 genotype, only 20% of these patients will develop kidney disease.12 This observation has led to the development of the “second hit” hypothesis, where environmental factors lead to increased APOL1 risk variant expression in podocytes. One factor associated with the development of APOL1 mediated kidney disease (AMKD) is high interferon states, especially due to viral infections such as human immunodeficiency virus (HIV), Parvovirus B12, or SARS-CoV-2.13
Other possible second hits include genetic etiologies, such as rare duplications of APOL1, and deletion mutations in APOL3, which physically interacts with APOL1, have also been linked to nephropathy development; inflammatory disease processes such as Systemic Lupus Erythematosus (SLE) are also associated with a greater risk of ESKD in patients with high-risk genotypes (Figure 3).14
While some “second hits,” such as high interferon states, are thought to lead to the development of kidney disease, other modifiers are protective. Recent studies have focused on a coding missense variant, p.N264K, which has been associated with a reduction in the risk of developing Focal Segmental Glomerulosclerosis (FSGS), CKD, and ESKD for carriers of the G2 risk allele.15 This exciting discovery provides another area of therapeutic focus and may help to reclassify some patients from being at high risk of kidney disease to a lower risk profile.
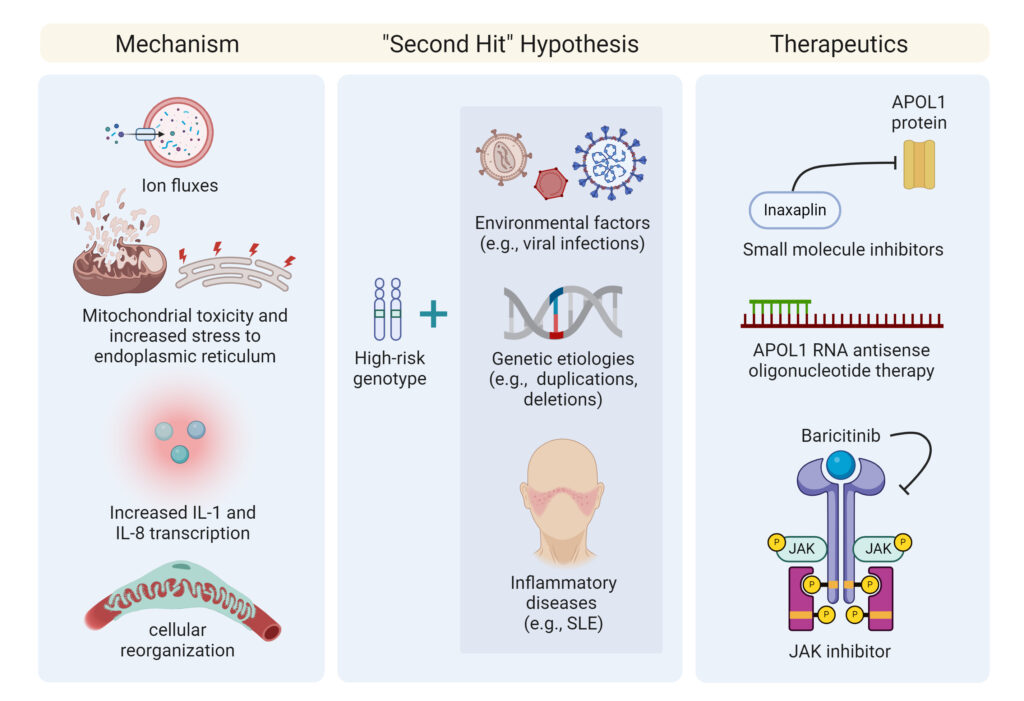
Figure 3: Postulated Mechanisms for Development of AMKD
Kidney Disease Phenotypes
A strong association between APOL1 and numerous kidney diseases has become evident. Some of the strongest associations has been between HIV-associated nephropathy (HIVAN) and a high-risk APOL1 genotype; in sub-Saharan Africa, this has led to an odds ratio of 87 and 29 in the United States.9 This strong association has also held true with FSGS with an odds ratio of 17; Kopp et al. also showed that FSGS associated with a high-risk APOL1 genotype was associated with a younger age of onset and faster rate of progression to ESKD.16 Additionally, Black patients with COVID-19 who developed collapsing glomerulopathies were all found to have high risk variants and no evidence of viral infection in the kidney on biopsy.17 High-risk APOL1 genotypes have also been associated with an increased risk of disease progression in other processes, such as lupus nephritis and sickle cell disease nephropathy, but do not appear to affect the risk of developing other glomerular diseases, such as IgA nephropathy (IgAN).2,18
Kidneys from deceased donors with a high-risk APOL1 genotype are associated with a higher rate of allograft failure even when accounting for other potential contributing factors.19 Additionally, living donors with 2 APOL1 risk variants are more likely to develop post-donation CKD.20 Although the recipient’s genotype has not been clearly shown to have an impact on the outcome of the allograft, a recent study by Zhang et al. reported that the number of recipient risk variant alleles was associated with an increased risk of death censored allograft loss independent of the donor’s genotype although this is yet to be confirmed in other large studies.21 The ongoing APOL1 Long-term Kidney Transplantation Outcomes study (APOLLO) will likely assist in further elucidating the relationship between recipient genotype and allograft outcomes.
Targeted Therapeutics
Given that plasma APOL1 does not appear to cause kidney disease phenotypes, clinical trials have primarily targeted the kidney directly. One of the most promising candidates is inaxaplin, a small molecule APOL1 channel inhibitor. Inaxaplin, in pre-clinical studies, blocked APOL1 channel function in vitro with a subsequent proteinuria reduction in mouse models. In a single group, open-label Phase 2a study, 16 participants with a high-risk APOL1 genotype, biopsy-proven FSGS and proteinuria (urine protein to creatinine ratio > 0.7 and < 10 g/g) received daily inaxaplin along with the standard of care for 13 weeks.22
All participants had an estimated glomerular filtration rate (eGFR) > 27 ml/min/1.73m2, and the primary outcome was a change in urine protein to creatinine ratio (UPCr) from baseline to week 13 in patients who were at least 80% adherent to the treatment regimen. The mean change in baseline to week 13 urine protein to creatinine ratio was -47.6%. Although 15 of the 16 participants experienced adverse events, these were either graded mild or moderate in severity, and none necessitated therapy discontinuation.22
The study is not without limitations, however, due to its small size and lack of a control group. Furthermore, treatment with renin-angiotensin-aldosterone system (RAAS) blockade was not maximized, and no patients were on sodium-glucose cotransporter-2 (SGLT2) inhibitors. The ongoing Phase 3 trial will help to further delineate the benefits of inaxaplin and a longer time period may help to delineate its impact on eGFR and not proteinuria alone.
Other investigational therapeutics are also under development as shown in Table 1. Antisense oligonucleotide therapy is in Phase 1 trials due to studies in mice showing a dose-dependent reduction in liver and kidney APOL1 mRNA.23 Activation of the janus kinase/signal transducer and activation of transcription (JAK/STAT) pathway is also felt to play a role in the development of kidney diseases, and a JAK inhibitor, baricitinib, is currently in early-stage investigation as another therapeutic for AMKD.23 The discovery of the coding variant, APOL1 p.N264K, provides another potential therapeutic target for an inhibitor that can mimic this coding variant and mitigate the development of AMKD.24
Table 1: Recent AMKD Clinical Trials
Genetic Testing
To identify patients with high-risk alleles, nephrologists will need to be comfortable with conducting genetic testing on a routine basis. Barriers to widespread adoption of genetic testing include a lack of perceived benefit, difficulties in interpreting genetic variants, cost, and the need for genetic counseling.25 Given historical injustices towards African Americans and other minority groups in research, clinicians must ensure they are able to have conversations with their patients to overcome these areas of concern.26 Given the shortage of genetic counselors, a novel approach towards increasing cultural competency among nephrologists in the genetic evaluation of African American living donors using chatbots is currently underway.27 Additional innovative strategies are needed to ensure that genetic testing is easily accessible to all nephrologists while ensuring cultural competency and avoiding further racial injustices. We propose the following pathway (Figure 4) for nephrologists in countries where genetic testing for APOL1 high risk variants is easily accessible:
The geographical distribution of the high-risk APOL1 genotype has been proven to vary significantly even within Africa thus affecting the pretest probability of genetic testing, which is also affected by other variables like genetic admixture resulting from migration. A typical example in Africa is the genotypic frequency of the high risk APOL1 genotype in parts of Nigeria and Ghana, approximately 25% with significantly lower genotypic frequencies of the high-risk variant in Eastern African countries.2
This is only one of the many challenges genotypic screening in Africa poses. The uptake of routine testing for high-risk APOL1 genotypes in a low or middle income country, where health systems are already constrained by resource limitations, will likely improve once robust data is available to show a mitigation in the development of CKD and ESKD. This will also be further buttressed by emerging therapeutic interventions, which if effective, will further justify routine screening.
The impact of the high-risk genotype on kidney transplantation in this region cannot be underscored and the results of the APOLLO study are eagerly awaited to provide some guidance in this regard. The position paper from the recently held KDIGO APOL1 Kidney Disease Controversies Conference in Ghana, also provides incremental progress to our efforts to answer the many questions that remain about high-risk APOL1 genotypes and AMKD. To the best of our knowledge, there are no established and operational guidelines or workflows for APOL1 genetic testing in Africa at the moment outside the context of clinical research and clinical trials.
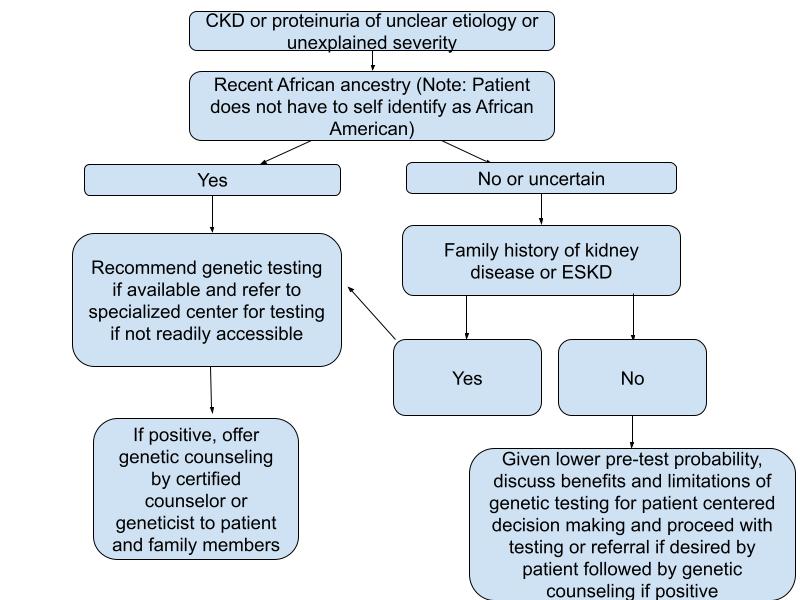
Figure 4: Clinical Approach for Genetic Testing
Future Directions
AMKD poses a significant global health burden; however, the exact mechanisms of nephrotoxicity remain uncertain. While some risk factors that serve as “second hits” have been identified, others remain unclear and under study. Nephrologists must be comfortable with performing genetic testing routinely as this is key to early identification and management of this disease process; further resources should be devoted to ensuring culturally competent genetic testing and the availability of genetic counseling for both patients and their family members especially in countries with an elevated high risk variant genotypic frequency. Physicians will need to have discussions with potential donors regarding pre-donation genetic testing and implications of donation if they are found to have a high risk genotype; the ongoing APOLLO study will assist with standardizing guidelines in this regard. Emerging therapeutics are on the horizon, and it is truly remarkable that a possible therapeutic for AMKD may be shortly available, given that high-risk variants were discovered less than 20 years ago.
References
1. United States Renal Data System. 2023 USRDS Annual Data Report: Epidemiology of kidney disease in the United States. National Institutes of Health, National Institute of Diabetes and Digestive and Kidney Diseases, Bethesda, MD, 2023.
2. Friedman DJ, Pollak MR. APOL1 Nephropathy: From Genetics to Clinical Applications. Clin J Am Soc Nephrol. 2021 Feb 8;16(2):294-303. doi: 10.2215/CJN.15161219. Epub 2020 Jul 2. PMID: 32616495; PMCID: PMC7863644.
3. Genovese G, Friedman DJ, Ross MD, Lecordier L, Uzureau P, Freedman BI, Bowden DW, Langefeld CD, Oleksyk TK, Uscinski Knob AL, Bernhardy AJ, Hicks PJ, Nelson GW, Vanhollebeke B, Winkler CA, Kopp JB, Pays E, Pollak MR. Association of trypanolytic ApoL1 variants with kidney disease in African Americans. Science. 2010 Aug 13;329(5993):841-5. doi: 10.1126/science.1193032. Epub 2010 Jul 15. PMID: 20647424; PMCID: PMC2980843.
4. Friedman DJ, Pollak MR. APOL1 and Kidney Disease: From Genetics to Biology. Annu Rev Physiol. 2020 Feb 10;82:323-342. doi: 10.1146/annurev-physiol-021119-034345. Epub 2019 Nov 11. PMID: 31710572.
5. Friedman DJ, Pollak MR. Apolipoprotein L1 and Kidney Disease in African Americans. Trends Endocrinol Metab. 2016 Apr;27(4):204-215. doi: 10.1016/j.tem.2016.02.002. Epub 2016 Mar 3. PMID: 26947522; PMCID: PMC4811340.
6. Johnstone DB, Shegokar V, Nihalani D, Rathore YS, Mallik L, Ashish, Zare V, Ikizler HO, Powar R, Holzman LB. APOL1 null alleles from a rural village in India do not correlate with glomerulosclerosis. PLoS One. 2012;7(12):e51546. doi: 10.1371/journal.pone.0051546. Epub 2012 Dec 26. PMID: 23300552; PMCID: PMC3530541.
7. Aminu Abba Yusuf, Melanie A Govender, Jean-Tristan Brandenburg, Cheryl A Winkler, Kidney disease and APOL1, Human Molecular Genetics, Volume 30, Issue R1, 1 March 2021, Pages R129–R137, https://doi.org/10.1093/hmg/ddab024
8. Nadkarni GN, Gignoux CR, Sorokin EP, Daya M, Rahman R, Barnes KC, Wassel CL, Kenny EE. Worldwide Frequencies of APOL1 Renal Risk Variants. N Engl J Med. 2018 Dec 27;379(26):2571-2572. doi: 10.1056/NEJMc1800748. PMID: 30586505; PMCID: PMC6482949.
9. Daneshpajouhnejad, P., Kopp, J.B., Winkler, C.A. et al. The evolving story of apolipoprotein L1 nephropathy: the end of the beginning. Nat Rev Nephrol 18, 307–320 (2022). https://doi.org/10.1038/s41581-022-00538-3
10. Jha A, Kumar V, Haque S, Ayasolla K, Saha S, Lan X, Malhotra A, Saleem MA, Skorecki K, Singhal PC. Alterations in plasma membrane ion channel structures stimulate NLRP3 inflammasome activation in APOL1 risk milieu. FEBS J. 2020 May;287(10):2000-2022. doi: 10.1111/febs.15133. Epub 2019 Dec 2. PMID: 31714001; PMCID: PMC7298666.
11. Pays E. The Mechanism of Kidney Disease Due to APOL1 Risk Variants. J Am Soc Nephrol. 2020 Nov;31(11):2502-2505. doi: 10.1681/ASN.2020070954. Epub 2020 Sep 17. PMID: 32943491; PMCID: PMC7608952.
12. Dummer PD, Limou S, Rosenberg AZ, Heymann J, Nelson G, Winkler CA, Kopp JB. APOL1 Kidney Disease Risk Variants: An Evolving Landscape. Semin Nephrol. 2015 May;35(3):222-36. doi: 10.1016/j.semnephrol.2015.04.008. PMID: 26215860; PMCID: PMC4562465.
13. Beckerman P, Susztak K. APOL1: The Balance Imposed by Infection, Selection, and Kidney Disease. Trends Mol Med. 2018 Aug;24(8):682-695. doi: 10.1016/j.molmed.2018.05.008. Epub 2018 Jun 7. PMID: 29886044; PMCID: PMC6101980.
14. Pollak MR, Friedman DJ. APOL1 and APOL1-Associated Kidney Disease: A Common Disease, an Unusual Disease Gene – Proceedings of the Henry Shavelle Professorship. Glomerular Dis. 2023 Jan 25;3(1):75-87. doi: 10.1159/000529227. PMID: 37113494; PMCID: PMC10126737.
15. Gbadegesin R, Martinelli E, Gupta Y, et al. APOL1 Genotyping Is Incomplete without Testing for the Protective M1 Modifier p.N264K Variant. Glomerular Dis. 2024;4(1):43-48. Published 2024 Feb 20. doi:10.1159/000537948
16. Kopp JB, Nelson GW, Sampath K, et al. APOL1 genetic variants in focal segmental glomerulosclerosis and HIV-associated nephropathy. J Am Soc Nephrol. 2011;22(11):2129-2137. doi:10.1681/ASN.2011040388
17.Wu H, Larsen CP, Hernandez-Arroyo CF, et al. AKI and Collapsing Glomerulopathy Associated with COVID-19 and APOL1 High-Risk Genotype. J Am Soc Nephrol. 2020;31(8):1688-1695. doi:10.1681/ASN.2020050558
18. Gbadegesin R, Lane B. Inaxaplin for the treatment of APOL1-associated kidney disease. Nat Rev Nephrol. 2023;19(8):479-480. doi:10.1038/s41581-023-00721-0
19. Freedman BI, Julian BA, Pastan SO, et al. Apolipoprotein L1 gene variants in deceased organ donors are associated with renal allograft failure. Am J Transplant. 2015;15(6):1615-1622. doi:10.1111/ajt.13223
20. Mena-Gutierrez AM, Reeves-Daniel AM, Jay CL, Freedman BI. Practical Considerations for APOL1 Genotyping in the Living Kidney Donor Evaluation. Transplantation. 2020;104(1):27-32. doi:10.1097/TP.0000000000002933
21. Zhang Z, Sun Z, Fu J, et al. Recipient APOL1 risk alleles associate with death-censored renal allograft survival and rejection episodes. J Clin Invest. 2021;131(22):e146643. doi:10.1172/JCI146643
22. Egbuna O, Zimmerman B, Manos G, et al. Inaxaplin for Proteinuric Kidney Disease in Persons with Two APOL1 Variants. N Engl J Med. 2023;388(11):969-979. doi:10.1056/NEJMoa2202396
23. Vasquez-Rios G, De Cos M, Campbell KN. Novel Therapies in APOL1-Mediated Kidney Disease: From Molecular Pathways to Therapeutic Options. Kidney Int Rep. 2023;8(11):2226-2234. Published 2023 Aug 29. doi:10.1016/j.ekir.2023.08.028
24. Hung AM, Assimon VA, Chen HC, et al. Genetic Inhibition of APOL1 Pore-Forming Function Prevents APOL1-Mediated Kidney Disease. J Am Soc Nephrol. 2023;34(11):1889-1899. doi:10.1681/ASN.0000000000000219
25. Knoers N, Antignac C, Bergmann C, et al. Genetic testing in the diagnosis of chronic kidney disease: recommendations for clinical practice. Nephrol Dial Transplant. 2022;37(2):239-254. doi:10.1093/ndt/gfab218
26. Iltis AS, Rolf L, Yaeger L, Goodman MS, DuBois JM. Attitudes and beliefs regarding race-targeted genetic testing of Black people: A systematic review. J Genet Couns. 2023;32(2):435-461. doi:10.1002/jgc4.1653
27. Smith JD, Agrawal A, Wicklund C, et al. Implementation of a culturally competent APOL1 genetic testing programme into living donor evaluation: A two-site, non-randomised, pre-post trial design. BMJ Open. 2023;13(5):e067657. Published 2023 May 15. doi:10.1136/bmjopen-2022-067657
Acknowledgments: Figures 1, 2, and 3 were created using BioRender.com